Compare Insteon
There are a number of home automation technologies available in Australia and New Zealand - X10®, ZigBee® and Z-Wave® just to name a few.
So how does Insteon compare?
Quick comparison
Insteon is the most reliable and cost effective home automation technology available anywhere in the world. It is the only home automation standard that features dual mesh technology; this gives it a big advantage over its competitors. In fact, Insteon is the simplest, yet most powerful home automation system available.
![]() X10® |
![]() ZigBee® |
![]() Z-Wave® |
![]() Insteon® |
|
Dual Mesh (Powerline & Wireless RF) | No |
No |
No |
Yes |
Powerline Communication | Yes |
No |
No |
Yes |
Powerline Data Rate (bps) | 20bps |
-- |
-- |
2,400bps |
RF Wireless Communication | No |
Yes |
Yes |
Yes |
RF Wireless Data Rate (bps) | -- |
20,000bps |
9,600bps |
38,400bps |
Full Mesh Network Communication | No |
No |
No |
Yes |
Automatic Netwrok Enrollment | No |
No |
No |
Yes |
Maximum Devices Per Network | 256 |
65,536 |
232 |
16,777,216 |
All Devices are Peers | No |
No |
No |
Yes |
Command Acknowledgment | No |
Yes |
Yes |
Yes |
X10 Compatible | Yes |
No |
No |
Yes |
In-depth comparison
For the more technically minded, an extremely detailed comparison between Insteon and almost all other home automation technologies can be found in the Insteon Comparison White Paper. A word of warning, this document can get very technical (it may hurt the brain a little).
For a more detailed comparison between Insteon and the three other most popular home automation technologies available in Australia and New Zealand, please click on a heading below.
The Insteon Range
Controllers
Responders
Accessories
Insteon vs X10 +Insteon vs X10 -
At the low end, X10 powerline signaling technology has been around since the 1970s, but its early adoption is its limiting factor—it is too unreliable and inflexible to be useful today as an infrastructure home-control network. Although the X-10 Ltd. company and factory are no longer in business, there are still numerous legacy devices still in use.
How X10 Works
Invented in Scotland by Pico Electronics in 1975, X10 was a pioneering breakthrough for its time, but even though it employed one of the world's first custom integrated circuits, the technology of the 1970s put severe constraints on its design.
For example, there can only be 256 different X10 devices on a single powerline, because each X10 device can only be assigned one of 16 possible House Codes (A through P) and one of 16 possible Unit Codes (1 through 16).
Furthermore, X10 defines only 16 different Command Codes, but not all devices can respond to all X10 commands. The six most common X10 commands are On, Off, Dim, Bright, All Units Off and All Lights On.
X10 transmits one bit of information at each powerline zero crossing (every 8.33 milliseconds). A simple command-plus-address message contains 100 bits and takes 833 milliseconds to send (8/10 of a second).
A one-millisecond burst of 120 KHz carrier signifies a one bit and the absence of a carrier signifies a zero bit. An X10 message consists of a 4-bit Start Code followed by an 8-bit House Code followed by a 10-bit Key Code. Each message is sent twice, followed by 6 zero-crossings of silence before starting another message. (The silence interval can be omitted if certain X10 Commands, such as Bright or Dim, are being repeated.)
Insteon Compared with X10
Developed as it was in the 1970s, X10 is too limited to function as a command and control infrastructure network in the mass market. Not only is X10 powerline signaling dreadfully slow, but X10 communication has no built-in mechanism to verify that X10 messages got through—X10 is open loop. Although X10 did eventually define Status Request and Status Response Commands, very few X10 devices actually employ them and those that do take even more time to send and receive the extra messages.
Installing more than a few X10 devices in a network is not for the faint of heart. It usually requires blocking couplers, repeaters and filters—and then is highly likely to function properly for just a short while. X10 is fundamentally subject to false positives, both because of its signaling design but also of its limited addressing schema. Many X10 customers have lived through a neighbor inadvertently controlling products in their homes.
Insteon Uses Both Powerline and Radio Communications
Because Insteon powerline messages are repeated using radio and radio messages are repeated on the powerline, Insteon messaging is highly reliable while still using simple signaling methods on both the powerline and the radio. Dual-band products also naturally couple the electrical phases without the need for additional products.
Insteon Devices are Simulcasting Repeaters
Because Insteon devices simultaneously repeat each other's messages, adding more devices to an Insteon network adds more energy to the Insteon signal. Thus, as an Insteon network grows it becomes more reliable, while adding X10 devices weakens an X10 network.
Insteon is Closed Loop
All Insteon devices can both listen and talk and the Insteon protocol requires that all Insteon messages that are not broadcast be acknowledged.
Insteon is (Much) Faster than X10
At each powerline zero crossing (every 8.33 milliseconds), Insteon sends 24-bits of information, while X10 sends only ½ bit. Thus Insteon's raw signaling rate is faster than X10's by a factor of 48.
Insteon Has a Large Address and Command Space
With room for more than 16 million addresses and 65 thousand basic commands, Insteon is clearly in a different league compared to X10 with only 256 addresses and 16 commands.
Conclusions
Insteon is fundamentally more reliable than single-band technologies (such as X10, ZigBee and Z-Wave).
Insteon is fundamentally more interoperable than other technologies because of Statelink.
Insteon is brilliantly simple:
- Insteon uses both existing house wiring and the airwaves to carry messages, so each signaling method can be kept very basic because they back each other up.
- Insteon repeats messages by simulcasting, with each new device adding more energy to the Insteon signal, so an Insteon network becomes more robust and reliable as more devices are added.
- Insteon products are backwards- and forwards-compatible due to Statelink.
- Insteon is optimized for command and control, with firmware that runs on the smallest microcontrollers.
Although Insteon is simple, simplicity is not a limiting factor, because Insteon bridge devices can connect to all kinds of outside resources like computers, smartphones, the Internet and other networks in the home whenever needed. Networks of Insteon devices can evolve as the marketplace does.
Most other networking schemes are far more complicated than Insteon, but without significant compensating benefits in command and control applications. This is understandable, because none of them are dual-mesh and most of them are routed, so they had to trade cost and complexity for reliability and performance. The highest-performance networks, like WiFi, Bluetooth and HomePlug, do not compete with Insteon because they are intended for different purposes, such as computer networking, media streaming or cable replacement.
"Quality means doing it right when no one is looking."
Henry Ford (1863-1947)
For a more detailed analysis of Insteon vs other home automation technologies, please download the Insteon Comparison White Paper.
Insteon vs ZigBee +Insteon vs ZigBee -
Insteon vs ZigBee Comparison Table
ZigBee is a routed radio networking standard developed by a group of ZigBee Alliance members. ZigBee is the latest name in an effort begun in the mid 1990s originally called "HomeRF." Ratified in December 2004 and released to the public in June 2005, the ZigBee specification defines the Application/Framework and Network/Security layers built atop the pre-existing IEEE 802.15.4 radio standard. ZigBee was designed to be used in widely diverse of applications, ranging from battery-operated devices to commercial and industrial building management.
IEEE 802.15.4, which was completed in May 2003, defines a Low-Rate Wireless Personal Area Network (WPAN) that includes a direct sequence spread spectrum (DSSS) physical radio (PHY) layer and a media access control (MAC) software layer. Several chip vendors offer 802.14.4 radios that include microprocessors and 128K of onboard memory for the ZigBee stack. 802.15.4 radios also find uses in numerous non-ZigBee applications.
ZigBee's design goals were:
- A wireless network for industrial controls, medical devices, alarms, building automation and home automation;
- A self-organizing mesh network;
- A low data rate; and
- Low power consumption (greater than one-year battery life).
ZigBee has defined multiple kinds of devices, including:
- Network coordinators, one per network, at the root of the network tree;
- FFDs (full-function devices), which can be routers; and
- RFDs (reduced-function devices), which cannot be routers.
Only FFDs can form a mesh network, so ZigBee also defines a star network that can include RFDs at the edge of the network and a hybrid network called a cluster tree. Within these networks there can be beaconing or non-beaconing in order to support battery-operated devices that wake up intermittently.
ZigBee 802.15.4 radios can operate at 2.4 GHz worldwide or at 915 MHz in the U.S. and 868 MHz in Europe, although the two lower frequencies have not found market acceptance.
How ZigBee Works
ZigBee, so named because its messages zigzag around like a bee, is a routed radio-only network. ZigBee uses IEEE 802.15.4 spread spectrum radios, but with extensive additional software specified by the ZigBee Alliance.
IEEE 802.15.4 Radios
The IEEE 802.15.4 Low-Rate Wireless Personal Area Network (WPAN) standard, released in May 2003, defines the Physical (PHY) and Media Access Control (MAC) layers for Direct Sequence Spread Spectrum (DSSS) radios.
In a given market, these radios can operate at two distinct frequencies, 868 MHz and 2.4 GHz in Europe and 915 MHz and 2.4 GHz in the U.S. and some other countries. The data rate varies depending on the frequency.
In a home environment, lower frequencies typically propagate two or more times farther than 2.4 GHz due to less absorption by construction materials. However, the market has chosen to produce only 2.4GHz products. Furthermore, should the market develop 868/915 radios they will not be able to communicate with the 2.4GHz products.
At the PHY level, 802.15.4 radios can perform receiver energy detection, link quality indication and clear channel assessment.
The MAC layer supports 64,000 nodes per network. All devices must have an 8-byte IEEE address, but 2-byte short addresses can optionally be allocated during specified network association and dissociation procedures.
802.15.4 defines both contention-free and contention-based channel access methods. For contention-free communications, an optional guaranteed timeslot (GTS) can be used for high priority messages, but the radios normally use CSMA-CD (carrier sense multiple access with collision detect) for contention-based messaging.
Packets are variable size, with a maximum length of 128 bytes or 104 bytes maximum payload. For battery operation, 802.15.4 defines an optional super-frame that allows low-power devices to wake up periodically. Packet overhead (non-payload) data takes from 15 to 35 bytes, with an additional 7 bytes for optional security encryption.
Insteon Compared with ZigBee
There are several noteworthy differences between Insteon and ZigBee that have consequences in the home control marketplace. The main differences are that:
- Insteon is dual-mesh, ZigBee is radio only;
- Insteon propagates messages by simulcasting, ZigBee routes messages;
- Insteon messages are backwards and forwards compatible, ZigBee messages are specific to revision level and profile;
- Insteon devices are peers, ZigBee has full-function devices and reduced-function devices;
- Insteon requires no separate network enrollment step;
- Insteon uses FSK radios in one band per market, ZigBee uses DSSS in two bands per market.
Taken together, these distinctions entail higher cost and complexity for ZigBee radio. Granting that the elevated cost buys ZigBee radio devices with somewhat higher performance than Insteon radios, Insteon's dual-mesh architecture provides a powerline backup that ZigBee can't rely on when its radio-only messaging does fail.
On balance, ZigBee's cost and complexity make it better suited for large industrial and commercial networks, whereas Insteon is optimized for the home.
Insteon Uses Both Powerline and Radio Communications
Radio communication is far from 100% reliable, as cell phone and WiFi users are frequently reminded from firsthand experience. ZigBee's single media design is clearly sub-optimal to Insteon's dual-mesh design. Industry participants have come to recognize this fact. As such efforts are underway to resolve this deficiency by bridging to a powerline technology. These efforts will clearly lead to networking inefficiencies, additional hardware requirements and added costs. ZigBee's 802.15.4 radios, although sophisticated, are not perfect—sometimes a ZigBee radio message will not get through and then the only recourse is to retry. Insteon, however, has backup—the powerline can go where radio may be blocked. This means that Insteon radios can be simpler and lower cost than ZigBee radios and the software can occupy a smaller footprint while running on ultra-low-cost microcontrollers.
Insteon Uses Simulcasting Instead of Routing
Conventional wisdom has it that network protocols must prevent messages from clobbering one another at all costs, with the result that system designers often choose message routing as the best way to achieve a well-behaved network. While this may be true for high-speed networks, at the modest data rates Insteon requires for home command and control, more than one copy of a message can be sent in the same synchronized timeslot. Simulcasting of messages avoids any need for network controllers and routers and it has the added benefit that as more devices simulcast a message, more energy goes into sending that message, so the more likely it is for the message to get through.
Insteon is a Peer-to-Peer Network
Because of the complexity of the ZigBee software stack, chips that contain it along with 802.15.4 radios typically have 128K bytes of onboard memory and a relatively high-performance microprocessor core. In an attempt to reduce the overall cost of a network of ZigBee devices, ZigBee has defined full-function devices (FFDs) and reduced-function devices (RFDs), with multiple stack protocols and options to support the different kinds of devices. Each ZigBee network must have at least one FFD to act as a network coordinator. Any RFDs must be at the perimeter of the network defined by the network coordinator and there is some danger that such leaf RFDs will reconnect to a different coordinator after signal loss.
Insteon, thanks to the simplicity of simulcasting, does not have any of these problems. The full Insteon engine, which is the same for every Insteon device, takes up less than 3K bytes, leaving over 5K bytes for application code in an 8K byte microcontroller, more than enough for lamp dimmers and simple controllers.
Insteon Requires No Network Enrollment
With a unique I.D. number assigned to every device during manufacture, Insteon devices automatically join an Insteon network and help with simulcasting repeated messages as soon as they are powered up. With no need for a separate network enrollment step, users only need to link button pushes on a controller with functions on a controlled device. This straightforward setup can be accomplished by anyone using a simple Tap-Tap procedure or optionally using software.
Insteon Radio Is Simpler
Insteon uses proven FSK (frequency shift keying) radios at 921 MHz (in Australia and New Zealand), while ZigBee uses complex DSSS (direct sequence spread spectrum) radios in two bands per market. FSK receivers are more than twice as sensitive as DSSS radios while only requiring one-fourth the silicon.
Even though ZigBee is not the only protocol used by 802.15.4 radios on the market, most chip vendors are currently only offering 2.4 GHz radios containing the ZigBee stack. Products using 868 MHz in Europe and 915 MHz in the U.S. have not appeared in the marketplace.
Insteon uses a single frequency of 921 MHz, which has two advantages. 921 MHz propagates farther than 2.4 GHz in typical home environments, and WiFi, which already occupies the 2.4 GHz band at higher power, cannot interfere with Insteon, although it might cause problems with ZigBee.
"Product design is not just what it looks like and feels like. Design is how it works."
Steve Jobs (1955-2011)
Insteon vs ZigBee Comparison Table
![]() |
![]() |
||
Media | Radio only | Powerline and radio | |
Module types | Network coordinator (1 per network) Full-function device (FFD) Reduced-function device (RFD) |
All are peers | |
Message propagation | Routing tables | Simulcasting repeaters | |
Network topology | Partial routed mesh – only FFDs are repeaters Partial star – RFDs are star networked each to a single FFD |
Full mesh | |
RF interoperability | No, 915 MHz and 2.4 GHz | Yes, 921 MHz | |
Command interoperability | No, 8 profiles and revisions thereof | Yes, Statelink | |
RF data rate, bps | 20K instantaneous at 868 MHz 40K instantaneous at 915 MHz 250K instantaneous at 2.4 GHz |
38400 instantaneous | |
RF PHY | DSSS in two ISM bands | FSK in ISM Band | |
Powerline data rate, bps | None | 13,165 instantaneous PL 2,880 sustained PL 1,440 standard msg payload 1,698 extended msg payload 1,034 user data |
|
Powerline PHY | None | 131.65 KHz carrier BPSK |
|
X10 Compatible | No | Yes | |
Powerline phase bridging | No | RF or hardware | |
Acknowledged | Yes | Yes | |
Addressing | 64-bit IEEE address 16-bit short address (65,536 devices) |
24-bit pre-assigned module I.D. (16,777,216 Devices) |
|
Network enrollment | Part of installation procedure | Automatic | |
Groups | -- | Databases in modules | |
Commands | Application profiles | 2-byte (65,536) | |
Device types | Application profiles | 3-byte (16,777,216) | |
Global clock | Optional beaconing | Powerline zero crossing | |
Retries | Yes | Yes | |
Message length | Variable, 128 byte maximum | 15, 33 bytes | |
Collision avoidance | Yes | Yes | |
Triac control | -- | Yes | |
SRAM | -- | 256 bytes | |
Flash | 128K bytes typical | 4K x 14 bits (7K bytes) | |
EEPROM external | -- | 4K bytes | |
EEPROM internal | -- | 256 bytes | |
Watchdog | -- | Yes |
For a more detailed analysis of Insteon vs other home automation technologies, please download the Insteon Comparison White Paper.
Insteon vs Z-Wave +Insteon vs Z-Wave -
Insteon vs Z-Wave Comparison Table
Z-Wave® is a routed, radio-only network owned by Sigma Designs, Incorporated, of Milpitas, CA. A public company (NASDAQ: SIGM), Sigma Designs purchased Z-Wave from ZenSys of Denmark, the originator of Z-Wave, on December 18, 2008.
Z-Wave sends messages using radio alone. If for any reason radio messaging fails, Z-Wave can't rely on the powerline to get messages through. Therefore, Z-Wave's designers were obliged to give it a number of relatively complex features in order to make it as reliable as they could.
Most notably, Z-Wave routes its messages through the network using a Source Routing Algorithm (SRA). The SRA requires message initiator devices to know the arrangement of other devices in the network (the topology) so that they can compute the best route for messages to travel. Maintaining and distributing a network topology database is an intricate software task, especially when some devices in the network are mobile. Therefore, to keep costs down, Z-Wave defines different kinds of devices, with the lowest-cost devices, called slaves, unable to initiate messages.
In contrast, Insteon is a dual-mesh network, using both radio and powerline to back each other up. Insteon does not route messages—it simulcasts them in precise, synchronized timeslots. Simulcasting is so much simpler than routing that all Insteon devices can be low-cost peers, with no need for complex master controllers or simplified reduced-function devices.
How Z-Wave Works
Intended for wireless home control applications, Z-Wave radio networking is designed for relatively few nodes (232 maximum, but manufacturers recommend no more than 30-50) that communicate on average every 5 to 15 minutes. Z-Wave messages are variable length, with a payload averaging 4 to 6 bytes. Message latency requirements are relaxed to 200 milliseconds or more.
Z-Wave Physical Layer
Z-Wave radios use an unlicensed carrier frequency of 908.42 MHz in the U.S., 868.42 MHz in Europe, 919.82 MHz in Hong Kong and 921.42 MHz in Australia. Data is modulated onto the carrier at 9600 to 100,000 bps using GFSK (Gaussian frequency shift keying).
Z-Wave Messaging
The minimum length of a properly formatted Z-Wave message is 9 bytes, but a routed message requires 12 bytes plus repeater data plus the payload.
The message protocol includes routing, frame acknowledgement, collision avoidance with random back off and a frame checksum with retransmission if necessary.
The Z-Wave network is self-organizing and self-healing. To achieve self-organization, Z-Wave nodes have software that discovers the node's neighbors and informs the network's Static Update Controller (SUC) about them. A Source Routing Algorithm (SRA) in devices capable of initiating communication finds message pathways and generates routes based on a network topology database. Self-healing requires software to dynamically generate new routes around temporarily unavailable nodes. Moving nodes have software routines that can request new neighbor searches automatically. This software, which is part of the Z-Wave stack, resides in on-chip memory.
Z-Wave Network Setup
Since Z-Wave devices do not possess unique network addresses (I.D. numbers) when purchased, there must be a procedure whereby the network's Static Update Controller (SUC) assigns I.D. numbers to devices being installed on the network. Z-Wave specifies that network installation should be accomplished either centrally using some kind of installer tool or locally using the devices themselves. However, different Z-Wave device vendors have adopted different methods for achieving network installation locally and these are not always compatible.
Z-Wave Association Process
To support the creation of associations between buttons on a controller and actions of a controlled device, Z-Wave specifies that:
- The network must provide an Association Wizard,
- There must be a sanity check of requested associations and
- All nodes must be able to present their supported capabilities.
Software routines to support these features, to the extent that product developers choose to develop and include them, further enlarge memory requirements.
Z-Wave Chips
Sigma Designs offers Z-Wave modules with 64K bytes of flash memory and provision for additional external memory. The earlier 3102 series modules have been replaced with the 4101 and 4102 series using a faster signaling rate. All modules have an integrated FSK radio and an 8051 processor core.
Z-Wave Device Types
Managing the routing of messages on a network is complicated, especially if some devices in the network are mobile. Z-Wave uses source routing, so any device capable of initiating communication must know which routes are currently possible, choose the best route and then embed the routing information into the messages that it sends. Such routing algorithms have been well developed for a variety of networks, but they require a lot of code. Lots of code means lots of memory on a chip and therefore higher build costs for devices that use the chip.
Z-Wave chips have 32K or 64K bytes of flash memory, with provision for adding more memory externally. To reserve as much memory as possible for the application code in a Z-Wave device, the Z-Wave communications stack must be kept as small as possible. Therefore, Z-Wave defines a number of different device types that have varying capabilities and stack sizes. The main Z-Wave device categories are Controllers, Routing Slaves and Slaves.
Z-Wave controllers can initiate communication with all nodes and so they have the largest stack. The master controller, called a SUC (static update controller), performs network management, distributes network topology information to secondary controllers and supports central or local device enrollment. Mobile controllers use a portable controller stack that allows devices to request rediscovery of moving nodes. There can also be a SIS (SUC I.D. server), which can automatically distribute network topology information to multiple controllers, but that software usually runs on a PC. Unless there is a SIS in the Z-Wave network, users have to manually copy network topology data from the master controller to any secondary controllers in the network whenever they add or remove Z-Wave devices.
Routing Slaves can initiate communication with a subset of nodes using a smaller Z-Wave stack. They depend on the SUC for network topology information.
Slaves have the smallest stack and can only respond to communications.
Z-Wave also defines Installers for doing centralized network setup and Bridge devices for connecting to other kinds of networks.
By defining devices with reduced-functionality in order to minimize cost, Z-Wave has given up the simplicity of peer-to-peer networking. This tradeoff is understandable in response to the complexity of routing, but because different devices have different capabilities, users have to know more about how the network functions. Perhaps the most restrictive issue for users is the requirement for a single master controller in a Z-Wave network.
Insteon Compared with Z-Wave
The main differences between Insteon and Z-Wave are:
- Insteon is dual-mesh, Z-Wave is radio only;
- Insteon propagates messages by simulcasting, Z-Wave routes messages;
- Insteon uses backwards and forwards compatible Statelink commands, Z-Wave uses commands that must be understood by the responder;
- Insteon devices are peers, Z-Wave has network controllers and slaves; and
- Insteon requires no separate network enrollment step.
Insteon Uses Both Powerline and Radio Communications
Radio communication is far from 100% reliable, as cell phone and WiFi users are frequently reminded from firsthand experience. Z-Wave's single media design is clearly sub-optimal to Insteon's dual-mesh design. Z-Wave radios, because they use narrowband FSK (frequency shift keying) signaling, are considerably less sophisticated than cell phones or WiFi. Furthermore, because they share the unlicensed 900 MHz ISM (industrial, scientific and medical) band, they are required to transmit at low power. Metal in the home can block or reflect radio waves—installing radio wall switches in metal junction boxes can cause particular difficulties.
That is why Insteon is dual-mesh—if radio fails, powerline provides a backup and vice-versa. In combination, two independently simple, low-cost signaling methods can be much more reliable than sophisticated, high-cost methods employed on single media with no backup.
Because it is radio-only, Z-Wave had to resort to complex network self-organization, self-healing and routing procedures in an attempt to maximize reliability. Insteon, on the other hand, even though it employs the same sort of narrowband FSK radios in the 900 MHz range as Z-Wave, achieves greater overall reliability with far less complication, because it relies on dual-mesh powerline backup and repeated message simulcasting.
Insteon Uses Simulcasting Instead of Routing
Simulcasting is much simpler than routing and more robust because multiple devices simulcasting the same message add to the signal power.
Routing entails complexity. Devices that initiate communication using a source routing algorithm, which is what Z-Wave uses, must know the topology of the network. Maintaining and distributing a network topology database is not trivial, especially with mobile devices. To keep costs down, Z-Wave has defined different classes of devices, some of which cannot participate in routing.
Insteon is a Peer-to-Peer Network
Insteon devices are two-way simulcasting repeaters, which means they all handle Insteon messages in exactly the same way, with no need for network controllers or routers. A Z-Wave network, on the other hand, must contain a Static Update Controller (SUC) along with other kinds of devices, including slaves or routing slaves.
If you want more than one controller in your Z-Wave network and you don't have a SIS (SUC I.D. Server), you must choose a single controller as a master and use that controller alone for network maintenance. To set up your other controllers as secondaries you must go through an involved controller replication procedure. Thereafter, any time you add or delete network devices using your single master controller, you must repeat the entire controller replication process for all of your other controllers.
In contrast, Insteon lets you add or remove Insteon devices of any kind at any time, because no matter how a device appears to the user, to the Insteon network all devices are peers. Therefore, setting up Insteon devices requires far less customer knowledge and involvement.
Insteon Requires No Network Enrollment
As a consequence of routing using network controllers, Z-Wave requires that new devices be enrolled in the Z-Wave network before controller buttons can be associated with device functions. Some Z-Wave vendors have found ways to hide the network enrollment step from users by combining it with the control association procedure, but others have elected to keep the two procedures separate. This situation is confusing if consumers have Z-Wave products from different vendors who have implemented different setup methods.
With Insteon this problem doesn't arise because there is simply no need for network enrollment. There is no routing and there is no network controller. At the factory, Insteon devices are each given a unique I.D. number that serves as a permanent network address. All Insteon devices automatically become part of an Insteon network and start simulcasting repeated messages as soon as a customer powers them up.
"Everything should be made as simple as possible, but not simpler."
Albert Einstein (1879-1955)
Insteon vs Z-Wave Comparison Table
![]() |
![]() |
||
Media | Radio only | Powerline and radio | |
Module types | Controller Static controller (SUC) Slave Routing slave Enhanced slave Installer Bridge |
All are peers | |
Message propagation | Routing tables | Simulcasting repeaters | |
Network topology | Routed mesh | Full mesh | |
RF interoperability | Yes, 908.42 MHz | Yes, 921 MHz | |
Command interoperability | No, application and revision specific commands | Yes, Statelink | |
RF data rate, bps | 9600 instantaneous | 38400 instantaneous | |
RF PHY | FSK in ISM Band | FSK in ISM Band | |
Powerline data rate, bps | None | 13,165 instantaneous PL 2,880 sustained PL 1,440 standard msg payload 1,698 extended msg payload 1,034 user data |
|
Powerline PHY | None | 131.65 KHz carrier BPSK |
|
X10 Compatible | No | Yes | |
Powerline phase bridging | No | RF or hardware | |
Acknowledged | Yes | Yes | |
Addressing | 32-bit home I.D. 8-bit node I.D. (232 devices per network) |
24-bit pre-assigned module I.D. (16,777,216 Devices) |
|
Network enrollment | Part of installation procedure | Automatic | |
Groups | Controller database | Databases in modules | |
Commands | Command classes | 2-byte (65,536) | |
Device types | Device classes | 3-byte (16,777,216) | |
Global clock | No | Powerline zero crossing | |
Retries | Yes | Yes | |
Message length | Variable | 15, 33 bytes | |
Collision avoidance | Yes | Yes | |
Triac control | Yes | Yes | |
SRAM | 2048 bytes | 256 bytes | |
Flash | 32K bytes | 4K x 14 bits (7K bytes) | |
EEPROM external | Up to 24K bytes | 4K bytes | |
EEPROM internal | 0 | 256 bytes | |
Watchdog | Yes | Yes |
For a more detailed analysis of Insteon vs other home automation technologies, please download the Insteon Comparison White Paper.

Request more information
Please enter your details to receive more information about Insteon, and to be kept up to date about product development and releases.
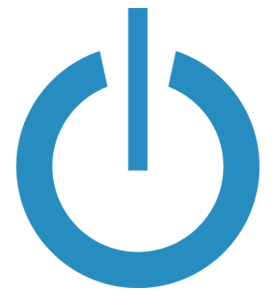

Find out more